History & Evolution
1890s: features in early research into acid production (Raistrick et al. 1919) | 1935: discovery of mechanism of action (Szent-Györgyi, A. et al., 1935) | 1937: description of role in Krebs cycle (Krebs et al. 1937)
Oxaloacetic acid , also known as oxalacetic acid, is a crystalline short-chain keto acid found in bacteria, plants and animals. Early research into oxaloacetic acid began in the 1890s and 1900s, during investigations into acid production in bacteria (Bentley, R., 1994). In 1919, Harold Raistrick, the “father of the study of fungal metabolites,” and Anne Clark hypothesized that oxaloacetic acid was a precursor of citrate in fungi (Raistrick et al. 1919). In 1935, Szent-Györgyi observed that C4dicarboxylic acids, including oxaloacetic acid, acted as catalysts in oxidation reactions (Szent-Györgyi, A. et al., 1935). Building on these findings, Hans Krebs and William Johnson investigated the role of citrate in carbohydrate metabolism, leading to the discovery of the “citric acid cycle” or Krebs cycle (also referred to as the tricarboxylic acid (TCA) cycle), in which oxaloacetic acid plays a key role (Krebs et al. 1937).
Since then, oxaloacetic acid has been found to participate in many other metabolic processes, including gluconeogenesis, the urea cycle, amino acid synthesis, and fatty acid synthesis (Wishart et al. 2022). It is therefore a metabolite of interest for a wide range of diseases, including cancer, diabetes, obesity and neurodegenerative conditions. Clinical trials involving oxaloacetic acid are quite rare, partly due to challenges in producing and storing the compound.
Biosynthesis vs. dietary uptake
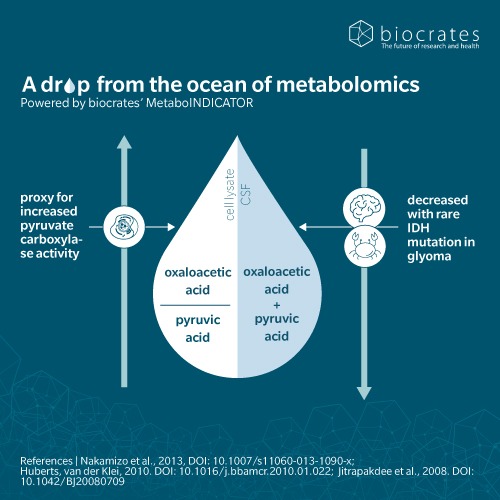
Oxaloacetic acid is synthesized endogenously in all living organisms. In humans and animals, this centers on the TCA cycle, which drives energy production in the mitochondria. Oxaloacetic acid is formed through the oxidation of malate, catalyzed by the enzyme malate dehydrogenase (Arnold et al. 2023). Citrate synthase then combines oxaloacetic acid with acetyl-CoA to form citrate. The next steps in the TCA cycle support the generation of reduced nicotinamide adenine dinucleotide (NADH), flavine adenine dinucleotide (FADH2) and adenosine triphosphate (ATP) (Icard et al. 2021). The production of oxaloacetic acid is the trigger for each “turn” of the TCA cycle, making it the rate-limiting substrate for the cycle (Arnold et al. 2023).
Oxaloacetic acid also plays a critical role in gluconeogenesis, where it is synthesized from pyruvate by pyruvate carboxylase in the mitochondria (Attwood et al. 1995). Interestingly, to continue along this pathway, oxaloacetic acid needs to be converted to a molecule that is more easily transported into the cytosol and then reconverted into oxaloacetic acid (Holecek 2023 ). Once this sub-cellular location issue is solved, the pathway can continue and oxaloacetic acid is converted to phosphoenolpyruvate (PEP) by the enzyme PEP carboxykinase following the reverse path of gluconeogenesis towards synthesis of glucose.
Plants and bacteria generate oxaloacetic acid via a variation of the TCA cycle, known as the glyoxylate cycle. In plants, oxaloacetic acid is synthesized in the mesophyll cells during the C4 acid cycle which underpins photosynthesis (Ludwig et al. 2016). It is quickly converted into malate or aspartate, and then transported to bundle-sheath cells in leaves, where decarboxylation releases CO2 for carbohydrate synthesis. In bacteria such as E. coli, oxaloacetic acid can be produced from PEP and is an essential precursor for succinate synthesis (Tan et al. 2013).
Oxaloacetic acid is found in several foods, including citrus fruits, wild rice, canola and peanuts (Wishart et al. 2022).
Oxaloacetic acid and the microbiome
Oxaloacetic acid plays a role in amino acid synthesis, particularly through transamination reactions using glutamate from the TCA and urea cycles (Shen et al. 2023). Oxaloacetic acid is directly involved in the synthesis of two non-essential amino acids, aspartate and asparagine. Aspartate is required for the synthesis of additional amino acids like methionine, lysine and threonine. The conversion of oxaloacetic acid to aspartate also generates fumarate, which is recycled into oxaloacetic acid via malate in the urea cycle.
The gut microbiome can heavily influence these processes by modulating the availability of amino acids and intermediates in the TCA and urea cycles. Oxaloacetic acid participates in bacterial fermentation in the gut, along with other TCA cycle intermediates including succinate, fumarate, citrate and malic acid (Louis et al. 2017). Gut bacteria also affect lipid metabolism by regulating the availability of acetyl-CoA, with oxaloacetic acid facilitating its transfer within cells for fatty acid synthesis.
Diet is relevant here, with research into low-carbohydrate and ketogenic diets highlighting the links between diet, microbiome and oxaloacetic acid (Longo et al. 2019). These diets reduce glucose and pyruvate availability, reducing oxaloacetic acid levels via the TCA cycle (Hartman et al. 2007). With less oxaloacetic acid, the body struggles to handle high levels of acetyl-CoA generated from fat, leading to ketone production as an alternative energy source. It has been suggested that oxaloacetic acid plays a role in the mechanism of action for the ketogenic diet’s beneficial effects on epileptic seizures (Hartman et al. 2007).
Oxaloacetic acid and neurological diseases
There is some evidence of an association between oxaloacetic acid and neurological disease, though findings are mixed and clinical trials in humans have not shown consistent benefits.
Animal studies suggest that oxaloacetic acid stimulates mitochondrial biogenesis in the brain, reduces neuroinflammation and promotes hippocampal neurogenesis (Wilkins et al. 2014). Several animal models of stroke have shown that oxaloacetic acid can improve neurologic performance and blood brain barrier integrity, and reduce lesion size (Campos et al. 2012). Oxaloacetic acid decreases blood glutamate levels, in turn reducing brain glutamate levels which are elevated in ischemic stroke. An animal model of amyotrophic lateral sclerosis (ALS) found that treatment with oxaloacetic acid preserved motor function, delayed onset of neurological symptoms and reduced neuroinflammation (Tungtur et al. 2021).
The glutamate-oxaloacetic acid link may also be relevant to potential treatments for Alzheimer’s disease (AD), Parkinson’s disease and epilepsy (Selivanov et al. 2021). The Trial of Oxaloacetic acid in Alzheimer’s Disease (TOAD) study looked at whether oxaloacetic acid could modify brain chemistry and metabolism in AD patients (Vidoni et al. 2021). However, while oxaloacetic acid was found to increase brain energy metabolism in higher doses, it did not lead to consistent changes in blood markers or improvements in cognitive scores.
A small proof-of-concept trial has shown that oxaloacetic acid treatment can significantly reduce fatigue in patients with myalgic encephalomyelitis/chronic fatigue syndrome and long COVID (Cash et al. 2022).
While these findings are encouraging, and patient trials have shown oxaloacetic acid treatment to be safe, more research is needed to validate the benefits observed in animal models.
Oxaloacetic acid, metabolic disorders and cardiovascular disease
Because of its role in cellular metabolism, gluconeogenesis and the TCA cycle, oxaloacetic acid is a metabolite of interest in the study of metabolic disorders and cardiovascular disease.
Changes in the TCA cycle have been linked to cardiovascular disease and metabolic syndrome (Calderón et al. 2013). A metabolomics study used gas chromatography-mass spectrometry to profile TCA metabolites, including oxaloacetic acid, in patients with coronary lesions, showing that risk factors like obesity, hypercholesterolemia and smoking affect TCA metabolite levels.
Oxaloacetic acid has been shown to activate the insulin signaling pathway in murine models (Wilkins et al. 2014). In humans, a 1968 study showed that oxaloacetic acid reduced hyperglycemia in type II diabetes (Yoshikawa et al. 1968), but more recent evidence is lacking. Oxaloacetic acid supplementation has been hypothesized to mimic calorie restriction, which may help to reduce fasting glucose levels and improve insulin resistance (Cash et al. 2009).
More recently, oxaloacetic acid has been shown to have a protective effect in liver disease and injury. A 2018 metabolomics study found oxaloacetic acid alleviated liver injury by scavenging reactive oxygen species, increasing ATP and inhibiting mitochondrial apoptosis (Kuang et al. 2018). Metabolomic profiling has also shown how gut microbiota may contribute to liver disease progression: a 2021 study found elevated concentrations of short chain fatty acids and their precursors, including oxaloacetic acid, in samples from non-alcoholic fatty liver disease-related hepatocellular carcinoma (Behary et al. 2021).
Oxaloacetic acid and cancer
Oxaloacetic acid is of particular interest in cancer research because of its ability to reverse the “Warburg effect,” which refers to the glycolytic processes used by cancer cells for energy generation (Samad et al. 2023). Oxaloacetic acid has been shown to inhibit lactate dehydrogenase A (LDHA) in cancer cells to reduce Warburg glycolysis, and thus impair cancer cell metabolism. Clinical studies show oxaloacetic acid can also decrease tumor development by blocking the conversion of glutamine to alpha-ketoglutarate and lowering NADPH levels.
A 2020 study used metabolomics to investigate metabolic changes in melanoma cells (Seo et al. 2020). Treatment with alpha-melanoma-stimulating hormone led to elevated levels of oxaloacetic acid and other TCA cycle metabolites, suggesting potential therapeutic targets for skin pigmentation inhibitors and anti-obesity drugs.
Beyond the lab, oxaloacetic acid supplementation has been approved by the US FDA for patients with glioblastoma multiforme and has been proposed as a safe adjunct treatment for glioma along with established chemotherapy protocols (Tucker et al. 2020).
Oxaloacetic acid and bone regeneration
A 2024 study reveals that oxaloacetic acid may be a promising option for bone regeneration therapies (Shirkoohi et al. 2024). This study found that oxaloacetic acid could be used to support the differentiation of stem cells into bone-forming cells, which may help to treat osteogenic disorders or injuries.
Given oxaloacetic acid’s central role in the TCA cycle, which is essential for numerous cellular processes – and the encouraging findings in multiple animal studies – there is a compelling case to further investigate this metabolite as a therapeutic candidate for a wide range of diseases.
References
Arnold, P. et al.: Regulation and function of the mammalian tricarboxylic acid cycle (2023) J Biol Chem | DOI: https://doi.org/10.1016/j.jbc.2022.102838
Attwood, P. et al.: The structure and the mechanism of action of pyruvate carboxylase (1995) Int J Biochem Cell Biol | DOI: https://doi.org/10.1016/1357-2725(94)00087-r
Behary, J. et al.: Gut microbiota impact on the peripheral immune response in non-alcoholic fatty liver disease related hepatocellular carcinoma (2021) Nat Commun | DOI: https://doi.org/10.1038/s41467-020-20422-7
Bentley, R. et al.: A history of the reaction between oxaloacetate and acetate for citrate biosynthesis: an unsung contribution to the tricarboxylic acid cycle (1994) Nat Commun | DOI: https://doi.org/10.1353/pbm.1994.0037
Calderón-Santiago, M. et al.: Method based on GC-MS to study the influence of tricarboxylic acid cycle metabolites on cardiovascular risk factors (2013) J Pharm Biomed Anal | DOI: https://doi.org/10.1016/j.jpba.2012.10.029
Campos, F. et al.: Oxaloacetate: a novel neuroprotective for acute ischemic stroke (2012) Int J Biochem Cell Biol | DOI: https://doi.org/10.1016/j.biocel.2011.11.003
Cash, A. et al.: Oxaloacetic Acid Supplementation as a Mimic of Calorie Restriction (2009) Open Longevity Science | DOI: http://dx.doi.org/10.2174/1876326X00903010022
Cash, A. et al.: Oxaloacetate treatment for mental and physical fatigue in myalgic encephalomyelitis/chronic fatigue syndrome (ME/CFS) and long-covid fatigue patients: a non-randomized controlled clinical trial (2022) J Transl Med | DOI: https://doi.org/10.1186/s12967-022-03488-3
Hartman, A. et al.: The neuropharmacology of the ketogenic diet (2007) Pediatr Neurol | DOI: https://doi.org/10.1016/j.pediatrneurol.2007.02.008
Icard, P. et al.: Understanding the central role of citrate in the metabolism of cancer cells and tumors: an update (2021) Int J Mol Sci | DOI: https://doi.org/10.3390/ijms22126587
Krebs, H. et al.: Metabolism of ketonic acids in animal tissues (1937) Biochem | DOI: https://doi.org/10.1042/bj0310645
Kuang, Y. et al.: Axaloacetate ameliorates chemical liver injury via oxidative stress reduction and enhancement of bioenergetic fluxes (2018) Int J Mol Sci | DOI: https://doi.org/10.3390/ijms19061626
Longo, R. et al.: Ketogenic diet: a new light shining on old but gold biochemistry (2019) Nutrients | DOI: https://doi.org/10.3390/nu11102497
Louis, P. et al.: Formation of propionate and butyrate by the human colonic microbiota (2017) Environ Microbiol | DOI: https://doi.org/10.1111/1462-2920.13589
Ludwig, M. et al.: The roles of organic acids in c4 photosynthesis (2016) Front. Plant Sci | DOI: https://doi.org/10.3389/fpls.2016.00647
Raistrick, H. et al.: On the mechanism of oxalic acid formation by aspergillus niger (1919) Biochem J | DOI: https://doi.org/10.1042/bj0130329
Samad, A. et al.: Oxaloacetate as a holy grail adjunctive treatment in gliomas: a revisit to metabolic pathway (2023) Cureus | DOI: https://doi.org/10.7759/cureus.48821
Selivanov, V. et al.: Unveiling a key role of oxaloacetate-glutamate interaction in regulation of respiration and ROS generation in nonsynaptic brain mitochondria using a kinetic model (2021) PLoS One | DOI: https://doi.org/10.1371/journal.pone.0255164
Seo, S. et al.: Metabolomics reveals the alteration of metabolic pathway by alpha-melanocyte-stimulating hormone in b16f10 melanoma cells (2020) Molecules | DOI: https://doi.org/10.3390/molecules25153384
Shen, F. et al.: Biochemistry, Amino Acid Synthesis and Degradation (2023) In StatPearls | DOI: https://www.ncbi.nlm.nih.gov/books/NBK559250/
Shirkoohi, F. et al.: Oxaloacetate as new inducer for osteogenic differentiation of human adipose tissue-derived mesenchymal stem cells in vitro (2024) Mol Biol Rep | DOI: https://doi.org/10.1007/s11033-024-09389-6
Szent-Györgyi, A. et al.: Über die Bedeutung der Fumarsäure für die tierische Gewebsatmung (1935) Hoppe-Seyler’s Zeitschrift für physiologische Chemie | DOI: ———
Tan, Z. et al.: Activating phosphoenolpyruvate carboxylase and phosphoenolpyruvate carboxykinase in combination for improvement of succinate production (2013) Appl Environ Microbiol | DOI: https://doi.org/10.1128/AEM.00826-13
Tucker, N. et al.: FDA Grants Fast Track Designation to Novel Molecule to Treat Glioblastoma (2020) Targeted Oncology | DOI: https://www.targetedonc.com/view/fda-grants-fast-track-designation-to-novel-molecule-to-treat-glioblastoma
Tungtur, S. et al.: Oxaloacetate treatment preserves motor function in SOD1G93A mice and normalizes select neuroinflammation-related parameters in the spinal cord (2021) Sci Rep | DOI: https://doi.org/10.1038/s41598-021-90438-6
Vidoni, E. et al.: Safety and target engagement profile of two oxaloacetate doses in Alzheimer’s patients (2021) Alzheimers Dement | DOI: https://doi.org/10.1002/alz.12156
Wilkins, H. et al.: Oxaloacetate activates brain mitochondrial biogenesis, enhances the insulin pathway, reduces inflammation and stimulates neurogenesis (2014) Human Molecular Genetics | DOI: https://doi.org/10.1093/hmg/ddu371
Wishart, D. et al.: Metabocard for oxalacetic acid (HMDB0000223) in HMDB 5.0: the human metabolome database for 2022 (2022) Nucleic Acids Res | DOI: https://hmdb.ca/metabolites/HMDB0000223
Yoshikawa, K. et al.: Studies on the anti-diabetic effect of sodium oxaloacetate (1968) Tohoku J Exp Med | DOI: https://doi.org/10.1620/tjem.96.127