History & Evolution
1784: discovery of citric acid (Berovic et al. 2007) | 1893: first synthesis (Wehmer 1893) | 1937: description of role in Krebs cycle (Krebs et al. 1937)
Citrate, the conjugate base of citric acid, is a versatile metabolite found in all aerobic organisms. Citric acid was first isolated from citrus fruits in 1784 by Swedish chemist Carl Wilhelm Scheele, who identified it as the source of the sour taste of lemons (Berovic et al. 2007). More than a hundred years later, in 1893, Carl Wehmer discovered that citrate could be produced from certain fungi (Wehmer, C., 1893). When the first world war disrupted the supply of calcium citrate, scientists like James Currie built on Wehmer’s research to find a new method of citrate production based on sugar fermentation (Currie 1917). The microbial production of citric acid using the mold Aspergillus niger would be patented in the early twentieth century, paving the way for industrial applications (Di Lorenzo et al. 2022).
Citrate attracted more attention in the 1930s, when Hans Krebs and William Johnson investigated the role of citrate in carbohydrate metabolism, leading to the discovery of the citric acid cycle, also known as the tricarboxylic acid (TCA) cycle or “Krebs cycle” (Krebs et al. 1937).
Today, citrate is recognized not only for its role in cellular energy production, but also for its involvement in various physiological processes such as fatty acid metabolism, amino acid synthesis, calcium signaling, anticoagulation and the regulation of pH balance. It has been found to play role in conditions such as kidney stones, bone disease and cancer.
As natural preservatives and sweeteners, citric acid and citrates are also commonly used in foods, drinks and supplements. Citric acid is the most consumed organic acid in the world, with more than 50% used in beverages (Granchi et al. 2019). Other uses include detergents, cosmetics, pharmaceuticals and industrial chemical applications. While citric acid derived from citrus fruits is considered safe, some people report adverse reactions when consuming processed foods containing synthetic citric acid, derived from modified strains of Aspergillus niger (Sweis et al. 2018).
Biosynthesis vs. dietary uptake
Citrate is obtained through the diet and synthesized endogenously. Dietary sources include fruits and vegetables – most obviously citrus fruits – and dietary supplements. Typical daily intake is around 4g, with over 95% absorbed in the small intestine (Granchi et al. 2019). Plasma citrate levels increase within 30 minutes of ingestion, and is then filtered and reabsorbed equally quickly by the kidneys (Granchi et al. 2019).
In addition to dietary intake, plasma citrate levels are influenced by renal clearance, cellular metabolism and bone remodeling (Granchi et al. 2019). Around 10 to 35% of filtered citrate is excreted in urine, varying by age, diet and sex (Costello et al. 2016) (Shah et al. 1958). Urinary citrate decreases with acidosis and testosterone administration, and increases with alkalosis and administration of estrogens, parathyroid hormone and vitamin D (Shah et al. 1958).
While diet is a good source of plasma citrate, it is not essential: humans and animals maintain normal citrate levels even with low levels of dietary citrate, indicating other sources (Costello et al. 2016).
All cells synthesize citrate through cellular metabolism in the TCA cycle. In this cycle, the enzyme citrate synthase catalyzes the condensation of oxaloacetate with acetyl coenzyme-A (acetyl-CoA) to form citrate. Citrate then acts as a substrate for a series of chemical reactions, generating reduced nicotinamide adenine dinucleotide, flavine adenine dinucleotide, adenosine triphosphate (ATP) and eventually regenerating oxaloacetate (Icard et al. 2021). Citrate transport protein releases any excess citrate from the mitochondria (Granchi et al. 2019). Through this mechanism, citrate plays a crucial role in energy production, fatty acid synthesis and bone formation.
Around 90% of total citrate in the body is stored in bone tissue (Costello et al. 2016). During bone resorption, citrate is released into plasma, providing the main endogenous source of circulating citrate.
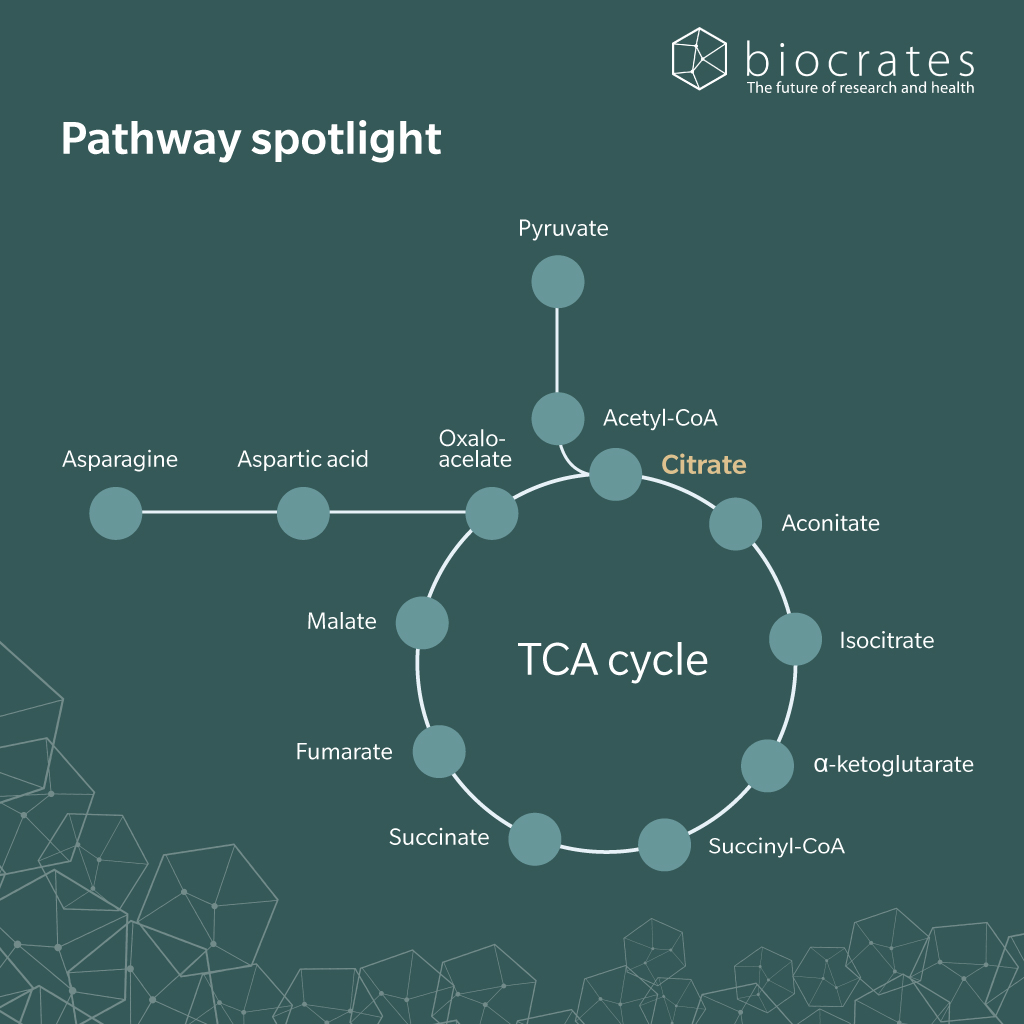
Citrate and the microbiome
The gut microbiome influences the availability of all metabolites involved in the TCA cycle, including citrate. Citrate participates in bacterial fermentation and contributes to regulation of acetyl-CoA, which influences lipid metabolism and fatty acid synthesis.
Citrate is a source of carbon and energy for several bacterial species (Bott 1997). In anaerobic conditions, citrate and other TCA intermediates are metabolized via citrate lyase by enterobacteria like Klebsiella pneumoniae and Escherichia coli, and lactic acid bacteria. This produces oxaloacetate, acetate and succinate, demonstrating the adaptability of these metabolic pathways under different environmental conditions.
A recent study in mice revealed several findings that suggest citric acid may be an alternative to antibiotics, which are increasingly overused (Hu 2024). Citric acid supplementation was shown to increase populations of common probiotic bacteria, such as Bifidobacteria and Lactobacillus. The study also found that citric acid promotes the expression of genes that maintain the integrity of the intestinal tight junction barrier, enhances intestinal immune function and inhibits viral infection.
Citric acid and immune signaling
In immune cells, citrate exported from mitochondria serves as a precursor for the synthesis of acetyl-CoA, which is essential for lipogenesis, histone acetylation, and antioxidant defenses (Zara et al. 2023). These processes not only sustain mitochondrial function but also link citrate to immune signaling and inflammation.
Studies have also linked citrate to the production of key inflammatory mediators, such as prostaglandins, nitric oxide and cytokines like TNF-α, IL-1β, and IL-6 (Liu et al. 2025). Furthermore, citrate regulates histone acetylation in immune cells through ATP citrate lyase, influencing the expression of genes involved in inflammatory pathways (Liu et al. 2025). These findings solidify the role of citrate in bridging metabolism, immune responses and inflammation.
Citric acid and bone disease
As noted, bone tissue serves as the main reservoir of citrate, giving it a central role in citrate homeostasis. At the same time, citrate is essential for bone health and skeletal integrity. Citrate is produced by osteoclasts and osteoblasts, but also influences their differentiation and behavior (Granchi et al. 2019). Disruptions to citrate homeostasis are associated with abnormalities in bone development and metabolism. Due to its impact on bone remodeling and resorption, citrate is a useful biomarker for orthopedic disorders (Liu et al. 2025).
Citrate has also been shown to be a promising treatment for osteoporosis. Research shows that dietary citrate increases bone mass in postmenopausal women with osteopenia (Jehle et al. 2013).
A metabolomics study investigating the relationship between citrate, bone formation and diabetes mellitus found that osteocytes increase citrate excretion in response to mechanical stress, which may promote bone formation (Villaseñor et al. 2019). This process was significantly impaired in high-glucose conditions.
Citric acid and urology
Alterations in renal clearance of citrate can lead to kidney dysfunction. In chronic kidney disease, the kidney’s ability to excrete and reabsorb citrate can be impaired, leading to lower levels of urinary citrate (Granchi et al. 2019). This increases the risk of calcium-containing kidney stones, as calcium is more likely to crystallize without citrate’s binding effect. Oral citrate therapy is commonly used to increase urinary citrate levels and help prevent and treat kidney stones, though evidence of its efficacy is unclear (Phillips et al. 2015).
A study combining metabolomics with kidney gene expression studies found that urinary excretion of citric acid cycle metabolites – particularly citrate – and renal expression of genes regulating these metabolites were reduced in non-diabetic CKD (Hallan et al. 2017).
Citric acid and neurology
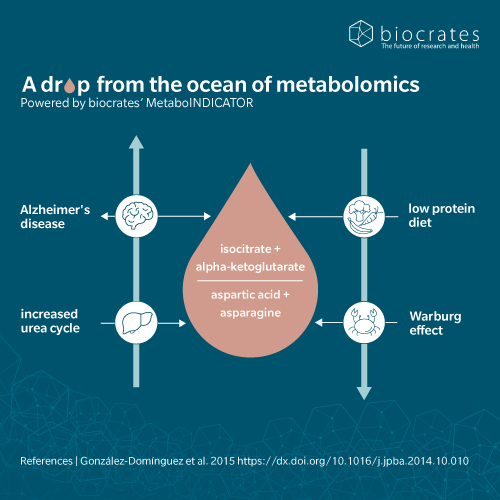
Citrate is attracting interest in the treatment of Alzheimer’s disease and dementia (AD) (Chhimpa et al. 2023). Low levels of citrate synthase and citrate impair the synthesis of acetyl-CoA and acetylcholine, resulting in loss of cognitive function in patients with AD. In addition, imbalances in the TCA cycle may contribute to the accumulation of amyloid-beta, which is implicated in AD. A recent network analysis suggesting that serum citrate concentrations are associated with cognitive decline (Jaramillo-Jimenez et al. 2023). This suggests that citrate may be a suitable biomarker and potential therapeutic option for AD and dementia.
The TCA cycle is also implicated in psychiatric conditions. A recent multiomics study using untargeted metabolomics, proteomics and DNA methylation data of patients with major psychiatric disorders confirmed the role of the TCA cycle metabolites, which may help identify biomarkers of disease (Hao M. et al. 2023).
Metabolomics research has also shown higher concentrations of citrate in the cerebrospinal fluid of patients with bipolar disorder compared to controls (Smedler et al. 2022).
Citric acid and oncology
Citrate is a major player in cancer cell metabolism, offering many potential avenues for cancer diagnosis and treatment. Citrate’s role in cancer metabolism was identified as part of the Warburg effect, where cancers preferentially use glycolysis even in the presence of oxygen (Icard et al. 2021). In proliferative cancer cells, low citrate levels sustain the Warburg effect by preventing inhibition of glycolytic enzymes. At the same time, citrate supports gluconeogenesis in cells relying on oxidative metabolism. Preclinical studies have shown that high doses of citrate have various anticancer effects, such as promoting apoptosis, neutralizing extracellular acidity and inhibiting tumor growth and signaling pathways (Icard et al. 2021). It may also enhance the effectiveness of cytotoxic drugs.
Citrate has been said to play a “dual role” in cancer, both supporting cancer cell proliferation and acting as a therapeutic agent (Icard et al. The dual role of citrate in cancer 2023). This opens the door to a “Trojan horse” therapeutic strategy, exploiting cancer cells’ reliance on citrate to disrupt their metabolic processes and trigger cell death (Petillo et al. 2020).
Citrate may be a useful biomarker for certain cancers: a review of multiomics approaches to metabolic phenotyping in prostate cancer puts citrate in the spotlight (Gómez-Cebrián et al. 2022). Citrate’s unique role in prostate cancer metabolism suggests it could be used as a biomarker alongside prostate-specific antigen (Galey et al. 2024).
Citric acid and 5P medicine
As shown above, multiomics approaches are providing a deeper understanding of citrate’s role in population health, disease prevention and treatment. These strategies are proving valuable not only in chronic conditions, but also in acute and emerging diseases.
For example, in the early stages of the COVID-19 pandemic, multiomics was used to provide deeper insights into the rapidly accumulating evidence and profile disease pathophysiology. This found notable citrate clusters, which led the researchers to conclude that monitoring and supplementing citrate levels could offer beneficial effects in severe COVID-19 patients (Overmyer et al. 2020).
References
Bott, M.: Anaerobic citrate metabolism and its regulation in enterobacteria (1997) Arch Microbiol | DOI: https://doi.org/10.1007/s002030050419
Chhimpa, N. et al.: The Novel Role of Mitochondrial Citrate Synthase and Citrate in the Pathophysiology of Alzheimer’s Disease (2023) J Alzheimers Dis | DOI: https://doi.org/10.3233/JAD-220514
Costello, L. and Franklin, R.: Plasma Citrate Homeostasis: How It Is Regulated; And Its Physiological and Clinical Implications (2016) HSOA J Hum Endocrinol
Currie, J.: The Citric Acid Fermentation of Aspergillus Niger (1917) J Biol Chem | DOI: https://doi.org/10.1016/S0021-9258(18)86708-4
Di Lorenzo, R. et al.: State of the Art on the Microbial Production of Industrially Relevant Organic Acids (2022) Catalysts | DOI: https://doi.org/10.3390/catal12020234
Galey, L. et al.: Rediscovering Citrate as a Biomarker for Prostate Cancer (2024) Nat Rev Urol | DOI: https://doi.org/10.1038/s41585-024-00899-3
Gómez-Cebrián, N. et al.: Metabolic Phenotyping in Prostate Cancer Using Multi-Omics Approaches (2022) Cancers (Basel) | DOI: https://doi.org/10.3390/cancers14030596
Granchi, D. et al.: Role of Citrate in Pathophysiology and Medical Management of Bone Diseases (2019) Nutrients | DOI: http://doi.org/10.3390/nu11112576
Hallan, S. et al.: Metabolomics and Gene Expression Analysis Reveal Down-Regulation of the Citric Acid (TCA) Cycle in Non-Diabetic CKD Patients (2017) eBioMedicine | DOI: https://doi.org/10.1016/j.ebiom.2017.10.027
Hao, M. et al.: Metabolome Subtyping Reveals Multi-Omics Characteristics and Biological Heterogeneity in Major Psychiatric Disorders (2023) Psychiatry Res | DOI: https://doi.org/10.1016/j.psychres.2023.115605
Hu, P. et al.: Citric Acid Promotes Immune Function by Modulating the Intestinal Barrier (2024) Int J Mol Sci | DOI: https://doi.org/10.3390/ijms25021239
Icard, P. et al.: Understanding the Central Role of Citrate in the Metabolism of Cancer Cells and Tumors: An Update (2021) Int J Mol Sci | DOI: https://doi.org/10.3390/ijms22126587
Icard, P. et al.: The Dual Role of Citrate in Cancer (2023) Biochim Biophys Acta Rev Cancer | DOI: https://doi.org/10.1016/j.bbcan.2023.188987
Jaramillo-Jimenez, A. et al.: Serum TCA Cycle Metabolites in Lewy Bodies Dementia and Alzheimer’s Disease: Network Analysis and Cognitive Prognosis (2023) Mitochondrion | DOI: https://doi.org/10.1016/j.mito.2023.05.002
Jehle, S. et al.: Effect of Potassium Citrate on Bone Density, Microarchitecture, and Fracture Risk in Healthy Older Adults Without Osteoporosis: A Randomized Controlled Trial (2013) J Clin Endocrinol Metab | DOI: https://doi.org/10.1210/jc.2012-3099
Krebs, H. et al.: Metabolism of Ketonic Acids in Animal Tissues (1937) Biochem J | DOI: https://doi.org/10.1042/bj0310645
Overmyer, K. et al.: Large-Scale Multi-Omic Analysis of COVID-19 Severity (2020) Cell Syst | DOI: https://doi.org/10.1016/j.cels.2020.10.003
Phillips, R. et al.: Citrate Salts for Preventing and Treating Calcium-Containing Kidney Stones in Adults (2015) Cochrane Database Syst Rev | DOI: https://doi.org/10.1002/14651858.CD010057.pub2
Smedler, E. et al.: Metabolomics Analysis of Cerebrospinal Fluid Suggests Citric Acid Cycle Aberrations in Bipolar Disorder (2022) Neurosci Appl | DOI: https://doi.org/10.1016/j.nsa.2022.100108