History & Evolution
1844: discovery (Gottlieb at al. 1844) | 1847: first synthesis (Dumas et al. 1847) | 1980s: elucidation of metabolic pathways
Propionic acid was first identified in 1844 by chemist Johann Gottlieb, during investigations into the effect of potassium hydroxide on sugar (Gottlieb et al. 1844). A few years later, French chemist Jean-Baptiste Dumas realized that other chemists had unknowingly produced the same compound by different means. He named the metabolite from the Greek words proto (“first”) and pion (“fat”), noticing that it was the smallest carboxylic fatty acid (Dumas et all. 1847). In 1878, Albert Fitz demonstrated bacterial synthesis of propionic acid, establishing the Fitz equation to describe its production by Propionibacteria during lactic acid fermentation (Bezirci et al. 2023). Subsequent research revealed antimicrobial and antifungal properties, leading to widespread use of propionic acid as a food preservative (Liu et al. 2012).
In recent decades, research has focused on propionic acid’s properties as a short chain fatty acid (SCFA) and its metabolic pathways in the human gut, highlighting the importance of microbial fermentation in energy homeostasis and overall health (den Besten et al. 2013). Propionic acid’s anti-inflammatory and anti-proliferative properties make it relevant in cancer research, and it plays a key role in lipid metabolism, neurological function, and as a biomarker for metabolic diseases such as obesity and diabetes (Zhang et al. 2023).
Propionic acid occurs naturally in humans, plants, microorganisms and animals. It has a wide range of industrial applications, including cheesemaking, preservatives, animal feed, pharmaceuticals, cosmetics and paints (Liu et al. 2012). It has a notoriously rancid smell, contributing to body odor and certain pungent cheeses (PubChem, 2025).
Biosynthesis vs. dietary uptake
In humans, propionic acid is derived from endogenous and environmental sources (Frye et al. 2016). Endogenous synthesis occurs during the catabolism of odd-chain fatty acids and branched-chain amino acids such as isoleucine and valine (Snyder et al. 2015). In these pathways, propionyl-CoA is formed and converted into methylmalonyl-CoA, which then is converted to succinyl-CoA and enters the TCA cycle.
Most propionic acid is synthesized by gut microbiota through the fermentation of dietary fibers (Louis et al. 2017). Microbial biosynthesis involves several distinct pathways in the gut, each characterized by different substrates and enzymatic reactions (Chen et al. 2025). These include:
- the succinate pathway, which converts succinate to propionate
- the acrylate pathway, which uses lactate and sugars as intermediates
- the propanediol pathway, which metabolizes deoxy sugars like rhamnose and fucose
- the dicarboxylic acid (Wood-Werkman) pathway, which involves the fermentation of pyruvate to propionate through intermediates such as oxaloacetate and methylmalonyl-CoA into propionic acid (Ranaei et al. 2020).
These pathways vary greatly depending on diet, microbiome diversity and host health (Chen et al. 2025).
Humans can also directly absorb propionic acid through the consumption of fermented foods. Although dietary propionic acid contributes modestly compared to microbial production, it still influences circulating plasma propionic acid levels (Chen et al. 2025). Diets rich in fermentable dietary fibers have been found to increase production of propionic acid by gut microbiota, while low-fiber diets are linked to decreased endogenous propionic acid synthesis and reduced plasma levels (Vinelli et al. 2022).
Once synthesized, PA is absorbed from the colon via the portal vein, with concentrations typically ranging between 100 and 300 µM, with around 6 μM in peripheral blood (Snyder et al. 2015). Most propionic acid is metabolized in the liver, leaving acetate as the most abundant SCFA in the periphery. Propionic acid is converted in the liver to propionyl-CoA, which can then be converted to pyruvate and used in energy metabolism and gluconeogenesis (Chen et al. 2025).
Plasma levels can also be affected by conditions like propionic acidemia, a rare inherited disorder, in which propionyl-CoA carboxylase deficiencies cause propionic acid to accumulate (Chen et al. 2025).
Elevated acetic acid-to-propionic acid ratios have been associated with obesity and insulin resistance (Müller et al. 2019). This is because acetic acid promotes lipogenesis and fat accumulation, while propionic acid acts as a counter-regulator, inhibiting fat synthesis and promoting glucose homeostasis. Thus, an imbalance where acetic acid dominates over propionic acid may result in increased fat storage and higher risk of developing metabolic syndrome.
Propionic acid and the microbiome
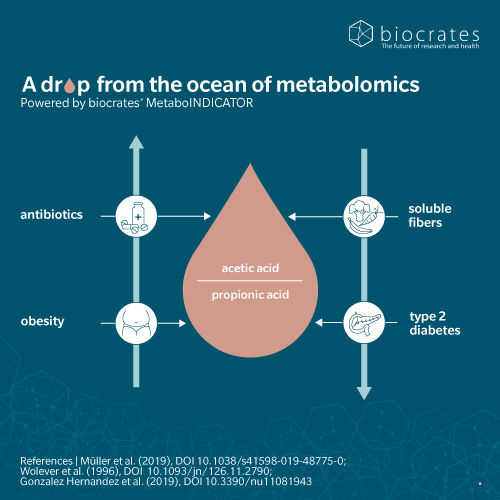
Different bacterial species contribute to the above pathways. For example, the succinate pathway is commonly found in Bacteroidetes and Firmicutes (Louis et al. 2017). The acrylate pathway operates in in Lachnospiraceae, and the propanediol pathway is observed in gut commensal bacteria including Roseburia inulinivorans and Blautia.
Alterations in microbiota can reduce propionic acid synthesis affecting gut integrity, immune function and metabolic processes (Zhang et al. 2023). For example, studies show that SCFA-producing bacteria are significantly reduced in patients with inflammatory bowel disease (IBD), and concentrations of propionate are reduced in the intestinal microbiome of these patients (Zhang et al. 2023), (Sun et al. 2017).
Propionic acid is also linked to the skin microbiome: species of Propionibacteria such as Cutibacterium acnes are found in the sebaceous glands, and are a recognized cause of acne (Bojar et al. 2004).
An animal model suggests that Bacteroides produces propionic acid in the gut, directly inhibiting the proliferation of the intestinal bacterial pathogen Salmonella (Jacobson et al. 2018).
Propionic acid and signaling
Like other SCFAs, propionic acid is an important signaling molecule involved in metabolic, immunological, neurological and cardiovascular processes (Zhang et al. 2023). This occurs primarily through activation of specific G protein-coupled receptors (GPCRs), particularly GPR41, affecting the pathways that regulate glucose homeostasis, immune responses and lipid metabolism (Zhang et al. 2023).
Propionic acid also modulates gene expression epigenetically by inhibiting histone deacetylases, affecting apoptosis, inflammation and immune function. Alongside acetic acid and butyric acid, propionic acid is an energy source for intestinal epithelial cells, promoting proliferation, differentiation and barrier integrity.
Dysregulation of propionic acid signaling pathways has been linked to metabolic disorders, neurodegenerative diseases, IBD and cardiovascular diseases, making propionic acid and related SCFAs promising therapeutic targets (Zhang et al. 2023).
Propionic acid and neurology
Propionic acid is increasingly recognized for its role in neurological health, particularly through the gut-brain axis (Killingsworth et al. 2021). Elevated propionate levels have been linked to behavioral and neurological changes observed in autism spectrum disorders (ASD), along with alterations in mitochondrial function, neuroinflammation, neurotransmitter imbalances and oxidative stress (MacFabe et al. 2012). Animal studies demonstrate that increased exposure to propionic acid can induce autism-like behaviors, underscoring its potential influence on neurodevelopment and cognition (Schulz et al.).
Abnormal regulation of propionic acid is also implicated in Alzheimer’s disease, where its role in inflammatory pathways and brain metabolism positions it both as a potential biomarker and therapeutic target (Killingsworth et al. 2021).
Propionic acid holds promise in neurodegenerative diseases such as Parkinson’s disease (PD) and multiple sclerosis (MS). In an animal model of PD, both depletion of dietary vitamin B12 (to induce propionic acid breakdown) and propionic acid supplementation were found to suppress neurodegeneration (Wang et al. 2024).
Clinical findings support this relationship, showing that decreased serum propionic acid levels correlated with more severe motor dysfunction and cognitive impairment in patients with PD (Wu et al. 2022).
Similarly, emerging metabolomic and clinical studies in MS suggest that propionic acid may exert beneficial immunoregulatory and neuroprotective effects (Lorefice et al. 2024) (Duscha et al. 2020). Propionate supplementation has been shown to boost regulatory T cells (Tregs), reduce pro-inflammatory Th1 and Th17 responses, and decrease brain atrophy, ultimately improving clinical outcomes in MS patients (Duscha et al. 2020). These findings underscore propionic acid’s potential as a complementary therapeutic strategy, although further clinical trials are needed to establish its efficacy and mechanisms.
Propionic acid and oncology
Recent studies point to the importance of propionic acid in oncology. Research in colorectal cancer indicates that propionic acid can inhibit tumor cell proliferation, potentially by inducing apoptosis and reducing inflammation (Young et al. 2022). In cervical cancer, propionic acid has been found to promote cell death by inducing mitochondrial dysfunction, apoptosis, autophagy and the accumulation of reactive oxygen species (Pham et al. 2021).
While more clinical trials are needed, these observations suggest that propionic acid holds potential as a biomarker of disease and therapeutic target. Encouragingly, propionic acid may be a suitable candidate for clinical trials, given that it is already approved for consumption as a food preservative.
Propionic acid and 5P medicine
Propionic acid is also worth investigating in the context of 5P medicine (preventive, predictive, precision, population-based, and participatory medicine). Propionic acid is of interest for its association with metabolic disorders such as obesity, insulin resistance and type 2 diabetes, suggesting a role in disease prevention and prognosis (Chambers et al. 2015). It has been shown to reduce obesity through activation of G-coupled protein receptors, leading to satiety, lower energy intake and weight loss (Chen et al. 2025). Given the association between obesity and other metabolic disorders, this suggests Propionic acid may be a good candidate for population-wide health interventions.
References
Bezirci, E. et al: Propionic acid production via two-step sequential repeated batch fermentations on whey and flour (2023) Biochemical Engineering Journal | DOI: https://doi.org/10.1016/j.bej.2023.108816
Bojar, R. et al: Acne and propionibacterium acnes Author links open overlay panel (2004) Clinics in Dermatology | DOI: https://doi.org/10.1016/j.clindermatol.2004.03.005
Chambers, E. et al: Effects of targeted delivery of propionate to the human colon on appetite regulation, body weight maintenance and adiposity in overweight adults (2015) Gut | DOI: https://doi.org/10.1136/gutjnl-2014-307913
Chen, X. et al: Elevated propionate and its association with neurological dysfunctions in propionic acidemia (2025) Front Mol Neurosci. | DOI: https://doi.org/10.3389/fnmol.2025.1499376
den Besten, G. et al: The role of short-chain fatty acids in the interplay between diet, gut microbiota, and host energy metabolism (2013) J Lipid Res. | DOI: https://doi.org/10.1194/jlr.R036012
Dumas, M. et al: Sur l’identité des acides métacétonique et butyro-acétique [On the identity of metacetonic acid and butyro-acetic acid] (1847) Comptes rendus
Duscha, A. et al: Propionic acid shapes the multiple sclerosis disease course by an immunomodulatory mechanism (2020) Cell | DOI: https://doi.org/10.1016/j.cell.2020.02.035
Frye, R. et al: Modulation of mitochondrial function by the microbiome metabolite propionic acid in autism and control cell lines (2016) Translational Psychiatry | DOI: https://doi.org/10.1038/tp.2016.189
Gottlieb, J. et al: On the effect of molten potassium hydroxide on raw sugar, rubber, starch powder, and mannitol (1844) Annalen der Chemie und Pharmacie
Jacobson, A. et al: A gut commensal-produced metabolite mediates colonization resistance to Salmonella infection (2018) Cell Host Microbe | DOI: https://doi.org/10.1016/j.chom.2018.07.002
Killingsworth, J. et al: Propionate and Alzheimer’s Disease (2021) Front. Aging Neurosci. | DOI: https://doi.org/10.3389/fnagi.2020.580001
Liu, L. et al: Microbial production of propionic acid from propionibacteria: current state, challenges and perspectives (2012) Crit Rev Biotechnol | DOI: https://doi.org/10.3109/07388551.2011.651428
Lorefice, L. et al: Propionic acid impact on multiple sclerosis: evidence and challenges (2024) Nutrients | DOI: https://doi.org/10.3390/nu16223887
Louis, P. et al: Formation of propionate and butyrate by the human colonic microbiota (2017) Environmental Microbiology | DOI: https://doi.org/10.1111/1462-2920.13589
MacFabe, D. et al: Short-chain fatty acid fermentation products of the gut microbiome: implications in autism spectrum disorders (2012) Microb Ecol Health Dis. | DOI: https://doi.org/10.3402/mehd.v23i0.19260
Müller, D. et al: Circulating but not faecal short-chain fatty acids are related to insulin sensitivity, lipolysis and GLP-1 concentrations in humans (2019) Scientific reports | DOI: https://doi.org/10.1038/s41598-019-48775-0
Pham, C. et al: Anticancer effects of propionic acid inducing cell death in cervical cancer cells (2021) Molecules | DOI: https://doi.org/10.3390/molecules26164951
Propionic acid-compound (2025) Pubchem | https://pubchem.ncbi.nlm.nih.gov/compound/Propionic-Acid
Ranaei, V. et al: Propionic acid: Method of production, current state and perspectives (2020) Food Technol Biotechnol | DOI: https://doi.org/10.17113/ftb.58.02.20.6356
Schulz, S. et al: Propionic acid animal model of autism (n.d.) Comprehensive Guide to Autism | DOI: https://doi.org/10.1007/978-1-4614-4788-7_106
Snyder, N. et al: Metabolism of propionic acid to a novel acyl-coenzyme A thioester by mammalian cell lines and platelets (2015) J Lipid Res. | DOI: https://doi.org/10.1194/jlr.M055384
Sun, M. et al: Microbiota metabolite short chain fatty acids, GPCR, and inflammatory bowel diseases (2017) Journal of Gastroenterology | DOI: https://doi.org/10.1007/s00535-016-1242-9
Vinelli, V. et al: Effects of dietary fibers on short-chain fatty acids and gut microbiota composition in healthy adults: A systematic review (2022) Nutrients | DOI: https://doi.org/10.3390/nu14132559
Wang, C. et al: Metabolic rescue of α-synuclein-induced neurodegeneration through propionate supplementation and intestine-neuron signaling in C. elegans (2024) Cell Reports | DOI: https://doi.org/10.1016/j.celrep.2024.113865
Wu, G. et al: Serum short-chain fatty acids and its correlation with motor and non-motor symptoms in Parkinson’s disease patients (2022) BMC Neurology | DOI: https://doi.org/10.1186/s12883-021-02544-7
Young, T. et al: Human gut-microbiome-derived propionate coordinates proteasomal degradation via HECTD2 upregulation to target EHMT2 in colorectal cancer (2022) The ISME Journal | DOI: https://doi.org/10.1186/s12964-023-01219-9
Zhang, D. et al: Short-chain fatty acids in diseases (2023) Cell Communication and Signaling | DOI: https://doi.org/10.1186/s12964-023-01219-9